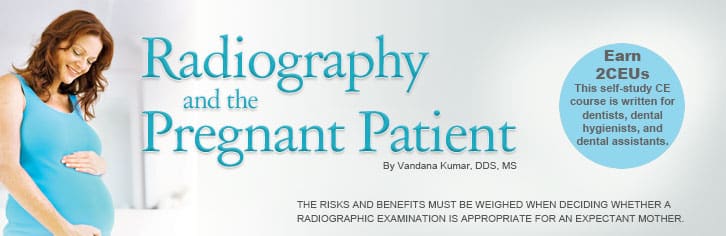
Radiography and the Pregnant Patient
The risks and benefits must be weighed when deciding whether a radiographic examination is appropriate for an expectant mother.
This course was published in the January 2013 issue and expires January 2016. The author has no commercial conflicts of interest to disclose. This 2 credit hour self-study activity is electronically mediated.
EDUCATIONAL OBJECTIVES
After reading this course, the participant should be able to:
Discuss the appropriateness of performing radiographs on pregnant women.
- Identify the basic concepts of ionizing radiation.
- List the risks of radiation exposure to a fetus.
- Detail strategies for reducing the risk of radiation exposure.
The use of diagnostic imaging in pregnant patients can be concerning for both patients and clinicians. Clinicians who have a solid understanding of the radiation doses delivered by various radiographic techniques and the acceptable exposure thresholds are best prepared to effectively advise their patients. Though radiographs can enhance diagnosis and treatment planning, the exposure to ionizing radiation may elevate a person’s lifetime risk of developing cancer. There are two principles of radiation protection to help manage the risk: appropriate justification for performing each procedure and careful optimization of the radiation dose used during each procedure. The latter concept is known as the principle of “as low as reasonably achievable” (ALARA).1 In other words, each patient should get the correct imaging exam, at the right time, with the appropriate radiation dose.
Determining whether a radiographic examination is appropriate for a pregnant woman remains controversial.2 Although a theoretical risk of carcinogenesis exists,3 there are no known risks of development/congenital malformations or mental retardation in a fetus exposed to ionizing radiation at the levels typically used for diagnostic imaging.4 The risk to the fetus has to be carefully weighed against the benefits of obtaining a radiograph, which is an essential component of a timely and comprehensive diagnosis.
CONCEPTS OF IONIZING RADIATION
X-rays and gamma rays are short, wavelength electromagnetic waves that cause ionization in tissues and can change cellular structures. Radiation effects are broadly categorized as stochastic or nonstochastic/deterministic. Stochastic effects result from cellular damage at the DNA level, which causes cancer or genetic mutations.5 Stochastic effects have no threshold value and can result from very low exposure, since their severity is independent of the radiation dose. Examples include cancer, leukemia, and inheritable genetic effects. Dental exposures can increase the risk of stochastic effects because they extend over the lifetime of the exposed individual.6
Nonstochastic/deterministic effects are caused by exposure to radiation at high doses. If the radiation dose is fractionated and distributed over time, an inherent cellular repair mechanism permits the tissue to recover from the damage. If the dose is administered all at once and exceeds the capability of innate cellular repair mechanism (threshold dose), the tissue is damaged.7 Conventional dental imaging does not cause deterministic effects.8 Radiation refers to the energy emitted by an ionizing radiation source. Radiation dose is the quantification of the ionizing radiation energy delivered to a given volume of tissue.9 Exposure is the total amount of ionization that is produced by a certain level of radiation per unit of mass of air. Absorbed dose is the energy absorbed per unit of mass by the body from a radiation exposure. Equivalent dose accounts for the relative biologic effects of different types of ionizing radiation (eg, particles and X-rays).
Effective dose incorporates how much of the body was exposed and the relative radiosensitivity of the exposed organs.10 It provides a useful standardized measurement for the biological effects of radiation because it considers not only the dose, but also the type, quantity, sensitivity, and carcinogenic potential of the irradiated tissue.11 The effective dose provides a measure of the cancer risk based on the nonuniform delivery of the radiation to the body.12 In terms of cancer induction, the most radiosensitive structures in the head and neck are the thyroid gland, parotid glands, bone marrow, and to a lesser extent, the esophagus. In diagnostic radiology, exposure is measured as coulombs/kg (C/kg); absorbed dose is measured as the unit, gray (Gy); and equivalent and effective doses are a measured by the unit, sievert (Sv). In diagnostic imaging—where the absorbed dose for a single radiographic exam is well below 1 Gy—mGy or mSv are used (1 Gy = 1,000 mGy and 1 Sv = 1,000 mSv).
Ionizing radiation has both teratogenic and carcinogenic effects on the developing fetus. The teratogenic risk of ionizing radiation is dose-dependent, a threshold below which effects do not appear. The teratogenic risk of fetal malformation increases significantly at doses above 150 mGy to 200 mGy and there is risk of fetal damage at exposures greater than 500 mGy.4,13
The carcinogenic risk of ionizing radiation is less established. There are many studies that link radiation to cancer formation of both solid tumors and leukemia.6,9 Analysis of these studies led to development of the linear nonthreshold hypothesis, which states that a linear relationship exists between dose and the risk of inducing cancer, and that all dose levels present a risk.14 This model extrapolates the risk associated with high doses (more than 100 mGy) where there are known effects in the low-dose range.15 The low-dose range is applied to clinicians who perform diagnostic radiology, workers in nuclear power plants and other industries, and individuals on long airline flights. Although some studies show no demonstrated cancer risk at doses lower than 100 mGy16 and patients may be harmed by avoiding diagnostic imaging due to false fear,17,18 the linear nonthreshold hypothesis is accepted worldwide for formulating radiation safety policies.19,20
Fetal risk is assessed by gestational age at exposure and the radiation dose.4,5,7,21 Fetal exposure is not the same as maternal exposure and an estimation of fetal exposure should be made in cases of maternal exposure.5 The fetus is considered directly exposed when it is located within the field of X-ray exposure. Indirect exposure of the fetus is caused by scatter from maternal tissues.5 Most estimates on fetal dose are full body (no attempt is made to isolate individual tissues and organs in a calculation of effective dose). Little data are available that demonstrate teratogenic or carcinogenic effects of radiation at levels experienced during dental X-rays. However, some qualitative observations can be made from the available data regarding fetal risk.21
GESTATIONAL AGE
At the point of early gestation and first trimester of the pregnancy, the rate of fetal development is characterized by intensive cell proliferation, differentiation, and migration, which are highly sensitive to radiation. The incidence of fetal death caused by radiation exposure in the first trimester is unknown because many women are not aware of the pregnancy at the time of the exposure or miscarriage. A fetal radiation dose of 50 mGy to 100 mGy during this period may either cause the failure of blastocyst implantation, resulting in spontaneous abortion, or cause no deterministic or stochastic effects at all because the cells of blastocyst are omnipotent and can replace damaged cells. Therefore, the radiation injury during early gestation has an all-or-nothing effect.
During the second trimester, the rapid growth rate of the fetus slows. However, the major organ systems are beginning to differentiate, which makes the developing fetus more vulnerable to radiation effects. Radiation exposure above 100 mGy at this stage could result in intrauterine growth retardation and central nervous system defects, such as microcephaly and mental retardation. The incidence of gross congenital malformations and mental retardation are dose-related and appear to have thresholds. In the third trimester, the fetus is less sensitive to radiation effects. Radiation at doses greater than 500 mGy during this period may deplete cell populations, but will not result in gross organ malformations. Table 1 provides a summary of radiation’s risk to the fetus related to dose.21
FETAL RISK
The maximum permissible radiation dose from naturally-occurring background exposure is 1 mSv per year. For a fetus, the average dose from naturally-occurring background radiation throughout a normal gestation period is 0.5 mSv to 1.0 mSv,13 which is less than the annual dose to the mother because some of the radiation is absorbed by maternal tissues.7 According to the National Council on Radiation Protection and Measurements (NCRPM), the maximum permissible radiation dose to the fetus of a pregnant radiation worker from occupational exposure is 0.5 mSv per month with the caveat that monitoring begins after the woman reports the pregnancy.22 The approximate fetal radiation exposure during most radiologic studies that use ionizing radiation is less than 0.05 Gy/50 mGy.13 NCRPM asserts that the risk of significant harm to the fetus is very low at 50 mGy and the risk of birth defects is increased only at doses above 150 mGy. Thus, the organization purports that spontaneous abortion caused by diagnostic procedures is rare.13
DOSE PER PROCEDURE
The radiation doses emitted from various dental diagnostic procedures pose almost negligible teratogenic risk to the fetus. Table 2 lists the effective doses (stated in microsieverts or ?Sv) for commonly used dental radiographic exams.23
The most widely used FMX technique in the United States includes the use of a round-aiming cylinder and D speed film. This technique carries more than a 20-per-million risk of death. Therefore, rectangular collimation and a high-speed film or digital sensor should be used to reduce the cancer risk by 10-fold.23 Maxillofacial cone beam computed tomographic (CBCT) imaging is used more commonly for 3D visualization of the craniofacial complex. CBCT produces views and volumetric reconstructions of craniofacial structures similar to multidetector CT but more quickly, at a lower cost, and at reduced radiation doses. Different CBCT machines can produce significant differences in radiation dose for the same examination.24
RADIATION DOSE REDUCTION
Affordable additions to all radiographic procedures can increase safety and reduce risk. Thyroid collars and protective aprons should be used to reduce exposure to radiosensitive organs and a developing fetus.18 If thinly layered bismuth radioprotective latex or leaded garments are used to shield the mother’s radiosensitive organs, the radiation dose reaching the fetus can be cut by 50%.25 The use of leaded glasses reduces radiation dose to the eye by 62% for a full FOV CBCT scan and 38% for a collimated CBCT scan. They should be worn by patients during all CBCT scanning procedures (both limited-volume scans that do not include the eyes and full-head scans) to limit the radiation absorbed by the lens of the eye and to reduce the risk of radiation cataract development.26 Table 3 provides a list of strategies for reducing radiation exposure produced by dental X-rays.
CONCLUSION
The teratogenic effects of ionizing radiation on the developing fetus are dose dependent and have a well-defined threshold. The diagnostic doses of ionizing radiation during pregnancy do not increase the incidence of congenital malformation, intrauterine growth retardation, or abortion. The vast majority of dental diagnostic radiation procedures involve fetal radiation doses that are below the threshold of 50 mSv. Maternal exposure should not be confused with fetal exposure. Though immediate nonstochastic/deterministic effects require too high a threshold to be relevant for humans, potential delayed stochastic effects, such as the development of radiation-related cancer, have no threshold or minimum dose. Therefore, the principles of ALARA should be applied in order to minimize all unnecessary exposure. Until the linear effect theory regarding the risks from ionizing radiation is disproven, dental clinicians must consider the harmful potential of ionizing radiation and minimize radiation dosage by imaging wisely and gently. Unnecessary procedures, such as exposing bitewing images or panoramic radiographs at routine dental checkups, should be postponed until after the pregnancy is complete.
ACKNOWLEDGEMENTS
The author would like to thank John Ludlow, DDS, MS; Muralidhar Mupparapu, DMD, MDS; and Cynthia C. Amyot, BSDH, EdD, for their help with this manuscript.
-
- ICRP Publication 105. Radiation protection in medicine. 5. 2007;37:1–63
- Wieseler KM, Bhargava P, Kanal KM, Vaidya S,Stewart BK, Dighe MK. Imaging in pregnant patients: examination appropriateness. Radiographics. 2010;30:1215–1229.
- Brent RL. Saving lives and changing familyhistories: appropriate counseling of pregnant women and men and women of reproductive age,concerning the risk of diagnostic radiation exposures during and before pregnancy. Am J Obstet Gynecol. 2009;200:4–24.
- Patel SJ, Reede DL, Katz DS, Subramaniam R,Amorosa JK. Imaging the pregnant patient for nonobstetric conditions: algorithms and radiation dose considerations. Radiographics.2007;27:1705–1722.
- Ratnapalan S, Bentur Y, Koren G. “Doctor, will that x-ray harm my unborn child?” CMAJ.2008;179:1293–1296.
- National Research Council. Committee to AssessHealth Risks from Exposure to Low Level of IonizingRadiation. Health risks from exposure to low levels of ionizing radiation: BEIR VII Phase 2. Washington, DC:National Academies Press; 2006.
- Wang PI, Chong ST, Kielar AZ, et al. Imaging of pregnant and lactating patients: part 1, evidence based review and recommendations. AJR Am J Roentgenol. 2012;198:778–784.
- White SC, Mallya SM. Update on the biological effects of ionizing radiation, relative dose factors and radiation hygiene. Aust Dent J.2012;57(Suppl):2–8.
- Furlow B. Radiation protection in pediatric imaging. Radiol Technol. 2011;82:421-439.
- 1990 Recommendations of the InternationalCommission on Radiological Protection. Ann ICRP.1991;21:1–201.
- Garcia Silva MA, Wolf U, Heinicke F, Grundler K,Visser H, Hirsch E. Effective dosages for recording Veraviewe pocs dental panoramic images: analogfilm, digital, and panoramic scout for CBCT. Oral Surg Oral Med Oral Pathol Oral Radiol Endod.2008;106:571–577.
- Martin CJ. Effective dose: how should it be applied to medical exposures? Br J Radiol.2007;80:639–647.
- Wagner LK, Lester RG, Saldana LR. Exposure ofthe pregnant patient to diagnostic radiations: a guide to medical management.2nd ed. Madison, Wis:Medical Physics; 1997.
- Brenner DJ. Extrapolating radiation-induced-cancer risks from low doses to very low doses.Health Phys. 2009;97:505–509.
- Preston DL, Ron E, Tokuoka S, et al. Solid cancer incidence in atomic bomb survivors: 1958-1998. Radiat Res. 2007;168:1–64.
- Kellerer AM. Risk estimates for radiation-induced-cancer–the epidemiological evidence. Radiat Environ Biophys. 2000;39:17–24.
- Brenner DJ, Sachs RK. Estimating radiation induced cancer risks at very low doses: rationale for using a linear no-threshold approach. Radiat Environ Biophys. 2006;44:253–256.
- Tubiana M, Aurengo A, Averbeck D, Masse R.Recent reports on the effect of low doses of ionizing radiation and its dose-effect relationship. Radiat Environ Biophys. 2006;44:245–251.
- Preston RJ. Update on linear non-threshold-dose-response model and implications for diagnostic radiology procedures. Health Phys. 2008;95:541–546.
- International Commission on RadiologicalProtection. Biological and epidemiological information on health risks attributable to ionising radiation: a summary of judgements for the purposes of radiological protection of humans. Available at: www.icrp.org/docs/biology_icrp_foundat_doc_for_web_cons.pdf. Accessed December 6, 2012.
- Brent RL. Developmental Risks of RadiologicalProcedures Utilizing Ionizing Radiation During Pregnancy. In: Radiation Protection in Medicine:Contemporary Issues Proceedings of the Thirty-FifthAnnual Meeting of the National Council on Radiation Protection and Measurements. Arlington: NCRP; 1999.
- National Council on Radiation ProtectionMeasurements. Limitation of exposure to ionizing radiation: recommendations of the NationalCouncil on Radiation Protection and Measurements. Report No. 116. United States; 1993.
- Ludlow JB, Davies-Ludlow LE, White SC. Patientrisk related to common dental radiographic examinations: the impact of 2007 InternationalCommission on Radiological Protection recommendations regarding dose calculation. J Am Dent Assoc. 2008;139:1237–1243.
- Ludlow JB, Ivanovic M. Comparative dosimetry of dental CBCT devices and 64-slice CT for oral and maxillofacial radiology. Oral Surg Oral Med Oral Pathol Oral Radiol Endod. 2008;106:106–114.
- Rehani MM, Berry M. Radiation doses in computed tomography. The increasing doses of radiation need to be controlled. BMJ. 2000;320:593–594.
- Prins R, Dauer LT, Colosi DC, et al. Significant reduction in dental cone beam computed tomography (CBCT) eye dose through the use of leaded glasses. Oral Surg Oral Med Oral Pathol Oral Radiol Endod. 2011;112:502–507.
From Dimensions of Dental Hygiene. January 2013; 11(1): 56, 59–61.