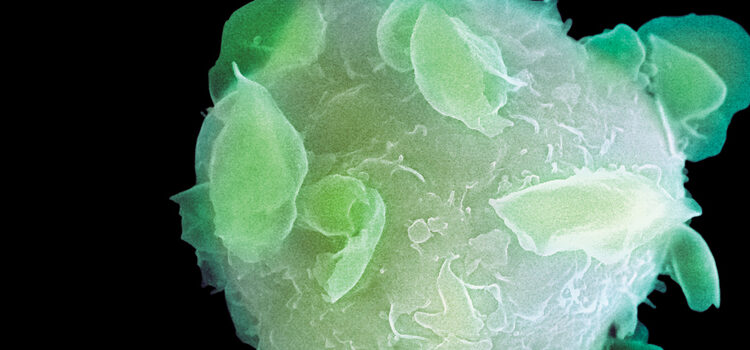
The Cell Revolution
How stem cells—particularly dental stem cells—may be used in tissue regeneration and repair.
Part 1 of 2: This is part one of a two-part series about the implications of stem cells for the dental professional
This course was published in the January 2009 issue and expires 1/31/12. The author has no commercial conflicts of interest to disclose. This 2 credit hour self-study activity is electronically mediated.
EDUCATIONAL OBJECTIVES
After reading this course, the participant should be able to:
- Identify various types of stem cells and their tissue sources found in the human body.
- Discuss the advantages of using adult stem cells versus embryonic stem cells in medical research.
- Describe the unique characteristics of stem cells recovered from human exfoliated deciduous teeth.
- Discuss the knowledge gained from research with adult dental stem cells for future medical therapies.
- Appreciate the value of using stem cells for personalized therapies in the developing field of regenerative medicine.
The recent discovery of stem cells in dental tissues has generated tremendous interest in both the lay and professional communities, as researchers explore the possibilities of using dental stem cells as a resource for tissue regeneration and repair. Numerous clinical trials are being conducted that seek to identify potential new medical treatments using stem cells.1 Stem cell-based therapies are being investigated for the treatment of many conditions, including neurodegenerative conditions; Parkinson’s disease; musculoskeletal disorders; autoimmune, liver, and cardiovascular diseases; diabetes; and for nerve regeneration following brain or spinal cord injury.1
Knowledge gained from this research contributes to the evolving field of “personalized medicine,” which targets specific medical therapies to individual patients. Therapies that use a patient’s own stem cells will allow for tissue regeneration and repair while minimizing risks for immune rejection that occur with donor tissues. Stem cell therapies will also include the use of genomic diagnostics and pharmacogenomic drug therapies, all of which tailor medical treatment to the individual. Scientific research supports the use of dental stem cells for future use in a multitude of regenerative therapies.2
Stem Cells
Stem cells are immature, unspecialized cells in the body that are able to differentiate into specialized cell types and tissues. Stem cells divide and grow rapidly and differentiate into other tissue types, including muscle, nerve, and components of the blood.1 See Figure 1.
When a stem cell divides, it divides into two daughter cells. Both daughter cells may remain unspecialized and are able to perpetually renew themselves through cell division.1,3 Self-renewing stem cells are able to go through numerous cycles of cell division—called passages—while maintaining their undifferentiated state, also known as stemness. Or, the daughter cells may differentiate into progenitor cells, which will eventually differentiate into a fully specialized cell type, usually from the tissue from which they were derived.1,3
Embryonic stem cells are the cells that make up the human body. At the fifth day following conception, an early stage embryo, called a blastocyst, is composed of 50 to 150 cells. Embryonic stem cells harvested from the blastocyst at this time are very immature, undifferentiated, and are pluripotent, meaning that they have the ability to grow into any cell type in the body.1,3 Immature pluripotent stem cells go on to differentiate into any of the three types of cells that derive the germ layers: ectoderm, endoderm, and mesoderm. Embryonic stem cells give rise to all 220 types of specialized cells that make up the human body.
As the embryo matures and parts of the body begin to emerge, progenitor stem cells within each future organ or tissue become further specialized. These stem cells are multipotent, meaning that they are capable of differentiating into multiple cell types within a certain embryonic germ layer.1,3 Multipotent stem cells form around day 14 of embryonic life, and are called adult stem cells. Eventually, the cells become incapable of further division, and differentiate into normal functional cells that compose the organs.1,3
A unique property of stems cells is their ability to expand naturally within the body (in vivo) and in growth culture medium outside of the body (in vitro). There are two primary sources of stem cells: embryonic stem cells, obtained from a 4- to 5-day old embryo, and adult stem cells, which are found dispersed in many organs and tissues in the human body and require recovery.1
Types of Stem Cells
Embryonic stem cells have the ability to differentiate into any cell type in the body. How – ever, ethical controversy exists around obtaining and using these stem cells for medical research and treatment purposes. Amniotic fluid-derived stem cells are also capable of proliferation and regeneration, but it is difficult and poses risk to the fetus to harvest stem cells through amniocentesis.1,4 A further disadvantage is that undifferentiated embryonic stem cells will continue to proliferate and grow unless controlled. This means that there is a risk for these cells to either grow into the wrong tissues or to form tumors known as teratomas.1
Currently, there are no approved treatments or human trials using embryonic stem cells. Twenty years of research have shown that embryonic stem cells pose great challenge for transplantation, including immune rejection, cellular malformation, and a tendency to produce teratomas and malignant carcinomas.1 Despite these limitations, embryonic stem cells remain a theoretically plausible source for regenerative therapies, due to their unlimited ability to/for replication and pluripotency. Many countries have placed legal restrictions on producing embryonic stem cells lines for research development and testing.1,5 Adult stem cells are found in most mature tissues, and are present in the fetus and in individuals after birth.6 Like all stem cells, adult stem cells can self-replicate and their ability to self-renew can last throughout the lifetime of individual organisms.1,3,6 But unlike embryonic stem cells, it is usually difficult to expand certain types of adult stem cells in culture.
Adult stem cells reside in specific organs and tissues, but account for a very small number of the total cells.1,6 These stem cells are buried deep within the tissues and are diffusely spread out, making recovery of these stem cells an invasive and expensive procedure in healthy individuals.
Adult stem cells can be found in bone marrow, blood, skin, dental pulp, retina, skeletal muscle, and brain.1,6 See Figure 2. Stem cells taken from bone marrow are hematopoietic and produce blood cells, or stromal (mesenchymal cells) and produce hard and soft tissues.1 Mesenchymal stem cells differentiate into fat cells and cells that compose bone, muscle, tendons, and connective tissue.1,6 Recently, mesenchymal cells have been found in dental pulp and supporting structures of the teeth, which turn into tooth-forming cells, and cells that repair oral soft tissues and bone.7
Previously, researchers believed that adult stem cells could only turn into cells that were the same as those in the tissues and organs in which they were found. Now, science has shown that adult stem cells taken from one area of the body can be transplanted into another area and grown into a completely different type of tissue. For example, research in animal models has found that mesenchymal stem cells can also help in the repair of cardiac muscle after acute myocardial infarction.8 The ability to differentiate into another type of tissue is called transdifferentiation or plasticity.1
Cells found in umbilical cord blood are unique in that they are rich in hematopoietic adult stem cells.9 They show great promise in their ability to differentiate into many different cell types, including liver, skeletal muscle, neural, and immune cells. Their advantage lies in the fact that they are more immature adult stem cells with pluripotent characteristics.9 However, there is only one opportunity to recover these stem cells for future medical use, when the mother has banked the baby’s cord blood after giving birth.
Dental Stem Cells
Mesenchymal stem cells are found in dental pulp, periodontal ligament, and the periosteum.10 An abundant source of these undifferentiated mesenchymal cells in permanent teeth is in the dental pulp of immature, impacted third molars.10
Mesenchymal stem cells have recently been discovered in the dental pulp of deciduous teeth, known as SHED (stem cells from human exfoliated deciduous teeth).11 SHED cells are formed in the developing embryo and demonstrate greater plasticity than stem cells taken from permanent teeth, meaning that they grow and multiply more rapidly as compared to those taken from the pulp of fully developed permanent teeth. These unique characteristics suggest that the immaturity of SHED cells shows greater potential for differentiation into a wider array of tissue types. Further, SHED cells are able to express proteins on their cell surfaces that allow them to not only differentiate into dental pulp, bone, and dentin, but also into neural and fat cells (adipocytes). In fact, SHED cells differentiate into nerve cells more readily than adult stem cells isolated from permanent teeth. SHED cells express a variety of neural growth factors and glial cell markers that directly reflect the embryonic neural crest origin of dental pulp and suggest that they may be important for use in future nerve regeneration therapies.11
Dental stem cells are the most accessible stem cells. They are isolated from many sources, including healthy dental pulp, periodontal ligament, bone, and the apical region of developing teeth, and can be recovered during a planned dental procedure.10 Stem cells within the pulp and at the apex of developing teeth are a valuable source of very proliferative, multipotent mesenchymal stem cells.12 Follicular tissue surrounding unerupted or impacted teeth is of ectomesenchymal origin and is also an excellent source for stem cells.11
Future Medical and Dental Stem Cell Therapies
The finding of stem cell plasticity carries significant implications for potential cell therapy. For example, if differentiation can be redirected, stem cells of abundant sources from easily accessible tissues, such as mesenchymal stem cells in bone marrow or stem cells within healthy dental pulp, could be used to repair tissues such as heart and nervous system tissue.8
This ability to regrow tissues is the focus of the field of regenerative medicine, which uses a patient’s own stem cells for biologically compatible therapies and individually tailored treatments. If needed, using a patient’s own stem cells (autologous donation) poses fewer risks for developing an immune reaction or graft rejection following transplantation, and avoids the risk of contracting diseases from another donor’s transplanted tissues.13
Recovered adult stem cells pose the least amount of ethical controversy and are the only type of stem cells currently being used in medical therapies. The first stem cell therapy was a bone marrow transplant, which has now been performed for more than 40 years in cancer treatment.14 New therapies include treatment for bone fracture and spinal fusion, and advances are being rapidly gained toward future treatments of neurodegenerative diseases, brain, and spinal cord injury.15 Given their ability to secrete many neurotropic factors, dental stem cells may hold promise for the regeneration of motor nerves following stroke or injury.16 Research findings also support that dental stem cells can promote the formation of new blood vessels (angiogenesis), which suggests an exciting future contribution to cardiovascular regeneration and repair.17
Adult stem cells may be used to regenerate bone and correct craniofacial defects.10 Both in vitro and in vivo research in animal models has shown that dental stem cells can regrow tooth roots, given a biological scaffold or trellis and the necessary growth factors. Results from animal studies imply that regenerated tooth roots are comparable to traditional surgical dental implants in terms of strength and function.18,19
SHED cells are capable of extensive proliferation and multipotent differentiation, which suggests their critical role in the regeneration and repair of dental and craniofacial defects and injuries. Recovered dental stem cells may one day be implanted into the pulp chambers of a severely traumatized or damaged tooth for nerve regeneration, which would revolutionize endodontic therapy.10 The periodontium is already capable of producing all of its surrounding tissues, and thus, periodontal stem cells may afford practitioners with an alternative supplemental therapy for treating the destruction caused by periodontal disease.10 Tissue-engineered bone grafts and joints will someday be available for a variety of surgical applications for dental specialists.10
Conclusions
Stem cell research is perhaps the most exciting and innovative path to revolutionizing the future of medicine. Despite tremendous gains in new knowledge and technology over the past decade, patients must still wait for years before new stem cell therapies will gain approval for use in this country. Some new therapies have been approved by the United States Food and Drug Administration, while others remain in review. Scientists are continuing their efforts to develop new technologies and products for future approval. Despite the uncertainties of the timeline for market approval, there is one strategy on which consumers should agree: recovering and storing stem cells now will ensure their availability in the future when they will be needed most. Part two of this article will explore how stem cells can be harvested from dental tissues and banked for future use.
Reference
- National Institutes of Health, US Department of Health and Human Services. Clinical Trials. Available at: http://clinicaltrials.gov/ct/search?term=stem+cell&submit=Search. Accessed December 10, 2008.
- Mao JJ. Stem cells and the future of dental care. New York State Dent J. 2008;74:20-24.
- Lakshmipathy U, Verfaillie C. Stem cell plasticity. Blood Rev. 2005;19:29-38.
- Barria E, Mikels A, Haas M. Maintenance and self-renewal of long-term reconstituting hematopoietic stem cells supported by amniotic fluid. Stem Cells Dev. 2004;13:548-562.
- National Institutes of Health, US Department of Health and Human Services. Stem Cell Information: Congressional Legislation. Available at: http://stemcells.nih.gov/policy/legislation.asp. Accessed December 10, 2008.
- Pittenger MF, Mackay AM, Beck SC, et al. Multilineage potential of adult human mesenchymal stem cells. Science. 1999;284:143-147.
- Gronthos S, Mankani M, Brahim J, Robey PG, Shi S. Postnatal human dental pulp stem cells (DPSCs) in vitro and in vivo. Proc Natl Acad Sci USA. 2000;97:13625-13630.
- Nagaya N, Fujii T, Iwase T, et al. Intravenous administration of mesenchymal stem cells improves cardiac function in rats with acute myocardial infarction through angiogenesis and myogenesis. Am J Physiol. 2004;287:H2670-H2676.
- Gang EJ, Jeong JA, Hong SH, et al. Skeletal myogenic differentiation of mesenchymal stem cells isolated from human umbilical cord blood. Stem Cells. 2004;22:617-624.
- Mao JJ, Giannobile WV, Helms JA, et al. Craniofacial tissue engineering by stem cells. J Dent Res. 2006;85:966-979.
- Miura M, Gronthos S, Zhao M, et al. SHED: Stem cells from human exfoliated deciduous teeth. Proc Natl Acad Sci USA. 2003;100:5807-5812.
- Gronthos S, Brahim J, Li W, et al. Stem cell properties of human dental pulp stem cells. J Dent Res. 2002;81:531-535.
- Barry FP, Murphy JM. Mesenchymal stem cells: clinical applications and biological characterization. Int J Biochem Cell Biol. 2004;36:568-584.
- Friedenstein AJ, Platetzky-Shapiro II, Petrakova KV. Osteogenesis in transplants of bone marrow cells. J Embryol Exp Morphol. 1966;16:381-390.
- Rahaman MN, Mao JJ. Stem cell based composite tissue constructs for regenerative medicine. Biotechnol Bioeng. 2005;91:261-284.
- Nosrat IV, Widenfalk J, Olson L, Nosrat CA. Dental pulp cells produce neurotrophic factors, interact with trigeminal neurons in vitro, and rescue motoneurons after spinal cord injury. Dev Biol. 2001;238:120-132.
- Gandia C, Armiñan A, Garcia-Verdugo JM, et al. Human dental pulp stem cells improve left ventricular function, induce angiogenesis, and reduce infarct size in rats with acute myocardial infarction. Stem Cells. 2008;26:638-645.
- Young CS, Abukawa H, Asrican R, Ravens M, Troulis MJ, et al. Tissue-engineered hybrid tooth and bone. Tissue Eng. 2005;11:1599-1610.
- Yu J, Shi J, Jin Y. Current approaches and challenges in making a bio-tooth. Tissue Eng Part B Rev. 2008;14:307-319.
From Dimensions of Dental Hygiene. January 2009; 7(1):30-33.