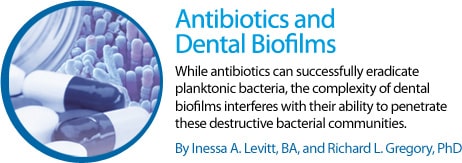
Antibiotics and Dental Biofilms
The complexity of dental biofilms interferes with the ability of antibiotics to penetrate these destructive bacterial communities.
This course was published in the January 2012 issue and expires January 2015. The authors have no commercial conflicts of interest to disclose. This 2 credit hour self-study activity is electronically mediated.
EDUCATIONAL OBJECTIVES
After reading this course, the participant should be able to:
- Discuss the formation of dental biofilms.
- Identify the differences between biofilm and planktonic bacteria.
- Explain how dental biofilms resist antibiotics.
Dental biofilms are formed when free-floating (planktonic) bacteria within saliva form a tight-knit structure that attaches to the tooth surface (Figure 1). Dental biofilms can contain more than 700 different bacterial species.1 Controlling dental biofilms remains a challenge for oral health care professionals. Although the mechanical removal of biofilm is effective, the use of antimicrobial chemicals to disturb and prevent biofilm formation may provide another option.
Numerous studies have demonstrated the effectiveness of antimicrobial agents on planktonic bacteria, however, the same conclusions cannot be applied to biofilms because of their unique nature.2,3 Biofilms’ ability to adhere to the tooth surface suppresses many genes—making dental biofilms different from planktonic bacteria, which only grow in a fluid environment, such as saliva. During the early stages of biofilm formation, planktonic cells attach to the tooth surface or bind to other bacterial cells using specific adhesins.3 This creates a structure that is stronger than its parts.4 The dispersal properties of biofilms allow them to spread and colonize to other available sites in the oral cavity.
Oral Biofilm Formation
The first step of biofilm formation requires the firm attachment of salivary glycoproteins or salivary pellicle to the tooth surface.3 This salivary pellicle is different from plaque, which is loosely bound to teeth. The pellicle is formed immediately after toothbrushing by salivary glycoproteins binding to the enamel. The salivary pellicle permits pioneer bacterial cells to attach to it. At this stage, the bacteria could change fates and become planktonic rather than acquire the framework of a biofilm. But, pioneer bacteria create an environment that is appealing to colonizing bacteria after the initial organisms take hold. These new bacteria are able to bind directly either to the pioneer bacteria or to the salivary pellicle. Later, colonizing bacteria react to the receptors on the pioneer bacteria by adhering to them. Various bacteria attach sequentially and create a more mature, potentially destructive biofilm.
This biofilm functions as a community of cells using a specialized mechanism called quorum sensing and spreads to nearby surfaces, in search of more nutrients—further harming the enamel.
Relationship Between Biofilm and Planktonic Bacteria
Bacteria find it more advantageous to function as biofilms.3 In working together, bacterial cells in a biofilm evolve and exhibit different mechanisms than they would as planktonic bacteria. Planktonic bacteria are often thought of as cells looking for a site on which to bind. For example, planktonic Streptococcus mutans has significantly more surface-bound antigen I/II—a pellicle-binding adhesin—than biofilm cells.5 In the oral cavity, biofilm cells are analogous to dental plaque while planktonic cells are the freefloating bacteria in saliva. (See “The Effects of Tobacco and Nicotine on Dental Biofilm and Caries” for a discussion of tobacco’s effects on oral bacteria).6
Resistance to Antibiotics
The accumulation of biofilm in the oral cavity makes it physically difficult for antimicrobial agents to penetrate a biofilm’s surface. Some studies have demonstrated that dental biofilms hinder the movement of antimicrobial agents to cells contained within the biofilm. Prevention of diffusion is not the only factor contributing to antibiotic resistance. The difference between the thickness and thinness of dental biofilms is also a consideration.7 Thick biofilms that produce catalase demonstrate more resistance to hydrogen peroxide than the same cells in thinner biofilms.7 Planktonic cells exhibit the least protection against antibiotics. Penetration of certain antimicrobial agents into biofilm could be weakened due to the matrix’s ability to consume the matter.
Biofilms are also able to release enzymes that degrade antibiotics.7 Because planktonic cells do not use quorum sensing and do not function together as a group, they are more susceptible to antibiotics. Cells within a biofilm are better protected against stresses, such as temperature change, changes in pH, and the addition of chemicals compared to planktonic cells. Biofilm cells are much more resistant to antimicrobial agents, such as antibiotics, than planktonic cells. Antibiotics commonly used in oral health care include amoxicillin, metronidazole, penicillin, tetracycline, and erythromycin.
Antibiotic resistance has become a worldwide problem in public health and is increasing among oral bacteria.8 The resistance of Streptococci to penicillin, amoxicillin, trimethoprim-sulfamethoxazole, and erythromycin has been observed in children receiving treatment for ear infections.9 Biofilms can protect bacteria from a challenging environment with several host defense mechanisms. The antigens of biofilm bacteria are hidden in the biofilm matrix and become less susceptible to the host immune system. Physical injury of the biofilm can also be reduced by the biofilm matrix. The short distance between bacterial cells makes cell-to-cell metabolic communications more frequent.10 The “insurance hypothesis” states that a single species is more vulnerable to the environment than multiple species. In other words, if there are more species in the biofilm and the structure of the biofilm is more complex and mature, its ability to resist antibiotics is greater.11 The antibiotic resistance of bacterial cells in biofilm is as much as 1,000 times to 1,500 times greater than the resistance of planktonic cells.12 Antibiotic resistance genes can be transferred between bacterial cells within biofilm—resulting in a biofilm-wide resistance to the antibiotic.13
The common carrier of antibiotic resistance genes are plasmids, which are circular double-stranded DNA species present mainly in bacteria (Figure 3). The replication of plasmids is independent of chromosomal DNA replication, and the number of plasmids in a cell varies widely. Plasmids with antibiotic-resistant genes are acquired from other cells by bacterial conjugation.
Sedlacek and Walker compared the effect of antibiotics on biofilm and planktonic cells of 19 subgingival bacterial species.14 Actinomyces naeslundii, Campylobacter, Fusobacterium nucleatum, Prevotella intermedia/nigrescens, P. loescheii, S. intermedius, S. parasanguis, S. sanguinis, Veillonella atypica, and V. parvula demonstrated significant differences in tetracycline, amoxicillin, and clindamycin resistance between planktonic and biofilm cells. In addition, antibiotic resistance can vary from one antibiotic to another. F. nucleatum growth was inhibited by 256 µg/ml, 128 µg/ml, and 64 µg/ml of tetracycline, amoxicillin, and clindamycin, respectively. Furthermore, resistance to a particular antibiotic can be different between bacterial species.
Effectiveness of Fluoride
Caries remains one of the most ubiquitous bacterial and infectious diseases, and the most effective anti-caries agent is fluoride.15 Van der Hoeven and Franken established that S. mutans -infected rats demonstrated significantly decreased levels of lactic acid in resting plaque when consuming fluoridated drinking water compared to a control group.16 Fluoride was not found to decrease the buildup of S. mutans , rather it decreased the acid produced from the bacterial cells. These findings indicate that fluoride can briskly penetrate biofilm.
Lactic acid is a major contributor to the demineralization of enamel. It is produced by lactate dehydrogenase (LDH)—an enzyme that converts pyruvic acid into lactic acid in the glycolytic cycle. When upregulated, LDH will cause S. mutans to produce greater amounts of lactic acid, decreasing pH levels (< 5.5), which allows bacteria to invade deep into the tooth, ultimately creating caries. Although fluoride is known to remineralize damaged enamel, its antibacterial effects could be broadened with the addition of another antimicrobial chemical. In a study by Tong et al, planktonic S. mutans survival rates were significantly reduced in the presence of nisin and sodium fluoride.17 Nisin is a bacteriocin that suppresses several types of Gram-positive organisms.
It is generally used as a food preservative to prevent spoilage by decreasing lactic acid production. The combination of sodium fluoride and nisin was more effective at disturbing biofilm than each agent alone.17
Conclusions
Promising treatments that may decrease the harmful consequences of biofilm buildup in the oral cavity are on the horizon. Antibiotic resistance in the oral cavity may be alleviated with new medications that address the prevalence of resistant biofilm bacteria.14 Further research is needed to decode the unknown mechanisms that contribute to the pervasiveness of biofilm.
PHOTO CREDIT
FIGURE 1: STEVE GSCHMEISSNER/SCIENCE PHOTO LIBRARY DAVID MACK/SCIENCE PHOTO LIBRARY
REFERENCES
- Aas JA, Paster BJ, Stokes LN, Olsen I, Dewhirst FE. Defining the normal bacterial flora of the oral cavity. J Clin Microbiol. 2005;43:5721-5732.
- Costerton JW, Lewandowski Z, Caldwell DE, Korber DR, Lappin-Scott HM. Microbial biofilms. Ann Rev Microbiol. 1995;49:711-745.
- Huang R, Li M, Gregory RL. Bacterial interactions in dental biofilm. Virulence. 2011;2:435-444.
- Hojo K, Nagaoka S, Ohshima T, Maeda N. Bacterial interactions in dental biofilm development. J Dent Res. 2009;88:982-990.
- Sanui T, Gregory RL. Analysis of Streptococcus mutans biofilm proteins recognized by salivary immunoglobulin A. Oral Microbiol Immunol. 2009;24:361-368.
- Allam E, Zhang W, Zheng C, Gregory RL, Windsor LJ. Smoking and oral health. In: Cigarette Smoke Toxicity—Linking Individual Chemicals to Human Diseases. Bernhard D, ed. Berlin: Wiley-VCH; 2011:257-280.
- Mah T, O’Toole G. Mechanisms of biofilm resistance to antimicrobial agents. Trends Microbiol. 2001;9:34-39.
- Kim SM, Kim HC, Lee SW. Characterization of antibiotic resistance determinants in oral biofilms. J Microbiol. 2011;49:595-602.
- Stewart PS. Mechanisms of antibiotic resistance in bacterial biofilms. Int J Med Microbiol. 2002;292:107-113.
- McCann KS. The diversity-stability debate. Nature. 2000;405:228-233
- Costerton JW. Introduction to biofilm. Int J Antimicrob Agents. 1999;11:217-221.
- Levy SB. The challenge of antibiotic resistance. Sci Ame. 1998;278:46-53.
- Erickson PR, Herzberg MC. Emergence of antibiotic resistant Streptococcus sanguis in dental plaque of children after frequent antibiotic therapy. Ped Dent. 1999;21:181-185.
- Sedlacek MJ, Walker C. Antibiotic resistance in an in vitro subgingival biofilm model. Oral Microbiol Immunol. 2007;22:333-339.
- Koo H. Strategies to enhance the biological effects of fluoride on dental biofilms. Adv Dent Res. 2008;20:17-21.
- Van der Hoeven JS, Franken H. Effect of fluoride on growth and acid production by Streptococcus mutans in dental plaque. Infect Immun. 1984;45:356-359.
- Tong Z, Zhou L, Jiang W, Kuang R, Li J, Tao R, Ni L. An in vitro synergetic evaluation of the use of nisin and sodium fluoride or chlorhexidine against Streptococcus mutans. Peptides. 2011;32:2021-2026.
From Dimensions of Dental Hygiene. January 2012; 10(1): 56-59.